A blog by YouTube Scientist Explorer Wanderer SonOfTerra92 https://www.youtube.com/user/SonOfTerra92
Tuesday, 9 July 2019
Sunday, 7 July 2019
NASA confirmed exoplanet table parameters and definitions
Database Column Name
|
Table Label
|
Description
|
|
---|---|---|---|
0 | pl_hostname | Host Star Name | Stellar name most commonly used in the literature. |
1 | pl_letter | Planet Letter | Letter assigned to the planetary component of a planetary system. |
2 | pl_name | Planet Name | Planet name most commonly used in the literature. |
3 | pl_discmethod | Discovery Method | Method by which the planet was first identified. |
4 | pl_controvflag | Controversial Flag | Flag indicating whether the confirmation status of a planet has been questioned in the published literature (1=yes, 0=no) |
5 | pl_pnum | Number of Planets in System | Number of planets in the planetary system. |
6 | pl_orbper | Orbital Period (days) | Time the planet takes to make a complete orbit around the host star or system. |
7 | pl_orbsmax | Orbit Semi-Major Axis (AU) | The longest radius of an elliptic orbit, or, for exoplanets detected via gravitational microlensing or direct imaging, the projected separation in the plane of the sky. |
8 | pl_orbeccen | Eccentricity | Amount by which the orbit of the planet deviates from a perfect circle. |
9 | pl_orbincl | Inclination (deg) | Angular distance of the orbital plane from the line of sight. |
10 | pl_bmassj | Planet Mass or M*sin(i) [Jupiter mass] | Best planet mass estimate available, in order of preference: Mass, M*sin(i)/sin(i), or M*sin(i), depending on availability, and measured in Jupiter masses. See Planet Mass M*sin(i) Provenance (pl_bmassprov) to determine which measure applies. |
11 | pl_bmassprov | Planet Mass or M*sin(i) Provenance | Provenance of the measurement of the best mass. Options are: Mass, M*sin(i)/sin(i), and M*sini. |
12 | pl_radj | Planet Radius (Jupiter radii) | Length of a line segment from the center of the planet to its surface, measured in units of radius of Jupiter. |
13 | pl_dens | Planet Density (g/cm**3) | Amount of mass per unit of volume of the planet. |
14 | pl_ttvflag | TTV Flag | Flag indicating if the planet orbit exhibits transit timing variations from another planet in the system (1=yes, 0=no).Note:Non-transiting planets discovered via the transit timing variations of another planet in the system will not have their TTV flag set, since they do not themselves demonstrate TTVs. |
15 | pl_kepflag | Kepler Field Flag | Flag indicating if the planetary system signature is present in data taken with the Kepler mission (1=yes, 0=no). |
16 | pl_k2flag | K2 Mission Flag | Flag indicating if the planetary system signature is present in data taken with the K2 Mission (1=yes, 0=no). |
17 | pl_nnotes | Number of Notes | Number of Notes associated with the planet. View all notes in the Confirmed Planet Overview page. |
18 | ra_str | RA (sexagesimal) | Right Ascension of the planetary system in sexagesimal format. |
19 | dec_str | Dec (sexagesimal) | Declination of the planetary system in sexagesimal notation. |
20 | ra | RA (decimal degrees) | Right Ascension of the planetary system in decimal degrees. |
21 | dec | Dec (decimal degrees) | Declination of the planetary system in decimal degrees. |
22 | st_dist | Distance (pc) | Distance to the planetary system in units of parsecs. |
23 | gaia_dist | GaiaDistance [pc] | Distance computed fromGaiaparallax. |
24 | st_optmag | Optical Magnitude [mag] | Brightness of the host star as measured using the V (Johnson) or the Kepler-band in units of magnitudes. |
25 | st_optband | Optical Magnitude Band | Band corresponding to the Optical Magnitude. Options are: V (Johnson) or Kepler-band. |
26 | gaia_gmag | G-band (Gaia) [mag] | Brightness of the host star as measuring using theGaiaband in units of magnitudes. Objects matched toGaiausing the Hipparcos or 2MASS IDs provided inGaiaDR2. |
27 | st_teff | Effective Temperature (K) | Temperature of the star as modeled by a black body emitting the same total amount of electromagnetic radiation. |
28 | st_mass | Stellar Mass (solar mass) | Amount of matter contained in the star, measured in units of masses of the Sun. |
29 | st_rad | Stellar Radius (solar radii) | Length of a line segment from the center of the star to its surface, measured in units of radius of the Sun. |
30 | rowupdate | Date of Last Update | Date of last update of the planet parameters. |
31 | pl_facility | Discovery Facility | Name of facility of planet discovery observations |
33 | pl_tranflag | Planet Transit Flag | Flag indicating if the planet transits its host star (1=yes, 0=no) |
34 | pl_rvflag | Planet RV Flag | Flag indicating if the planet host star exhibits radial velocity variations due to the planet (1=yes, 0=no) |
35 | pl_imgflag | Planet Imaging Flag | Flag indicating if the planet has been observed via imaging techniques (1=yes, 0=no) |
36 | pl_astflag | Planet Astrometry Flag | Flag indicating if the planet host star exhibits astrometrical variations due to the planet (1=yes, 0=no) |
37 | pl_omflag | Orbital Modulation Flag | Flag indicating whether the planet exhibits orbital modulations on the phase curve (1=yes, 0=no) |
38 | pl_cbflag | Circumbinary Flag | Flag indicating whether the planet orbits a binary system (1=yes, 0=no) |
39 | pl_angsep | Calculated Angular Separation [mas] | The calculated angular separation (semi-major axis/distance) between the star and the planet. This value is only calculated for systems with both a semi-major axis and a distance value. |
40 | pl_orbtper | Time of Periastron (Julian Days) | The time at which the orbiting body is at its closest approach to the star it orbits (i.e. is at periastron). |
41 | pl_orblper | Longitude of Periastron (deg) | The angular separation between the ascending node of the orbit and the location in the orbit of periastron. |
42 | pl_rvamp | Radial Velocity Amplitude [m/s] | Half the peak-to-peak amplitude of variability in the stellar radial velocity. |
43 | pl_eqt | Planet Equilibrium Temperature [K] | The equilibrium temperature of the planet as modeled by a black body heated only by its host star, or for directly imaged planets, the effective temperature of the planet required to match the measured luminosity if the planet were a black body. |
44 | pl_insol | Insolation Flux [Earth flux] | Insolation flux is another way to give the equilibrium temperature. It's given in units relative to those measured for the Earth from the Sun. |
45 | pl_massj | Planet Mass (Jupiter mass) | Amount of matter contained in the planet, measured in units of masses of Jupiter. |
46 | pl_msinij | Planet M*sin(i) (Jupiter mass) | Minimum mass of a planet as measured by radial velocity, measured in units of masses of Jupiter. |
47 | pl_masse | Planet Mass (Earth mass) | Amount of matter contained in the planet, measured in units of masses of the Earth. |
48 | pl_msinie | Planet M*sini(i) [Earth mass] | Minimum mass of a planet as measured by radial velocity, measured in units of masses of Earth. |
49 | pl_bmasse | Planet Mass or M*sin(i) [Earth mass] | Best planet mass estimate available, in order of preference: Mass, M*sin(i)/sin(i), or M*sin(i), depending on availability, and measured in Earth masses. See Planet Mass M*sin(i) Provenance (pl_bmassprov) to determine which measure applies. |
50 | pl_rade | Planet Radius (Earth radii) | Length of a line segment from the center of the planet to its surface, measured in units of radius of the Earth. |
51 | pl_rads | Planet Radius (solar) | Length of a line segment from the center of the planet to its surface, measured in units of radius of the Sun. |
52 | pl_trandep | Transit Depth (percentage) | The size of the relative flux decrement caused by the orbiting body transiting in front of the star. |
53 | pl_trandur | Transit Duration (days) | The length of time from the moment the planet begins to cross the stellar limb to the moment the planet finishes crossing the stellar limb. |
54 | pl_tranmid | Transit Midpoint (Julian days) | The time given by the average of the time the planet begins to cross the stellar limb and the time the planet finishes crossing the stellar limb. |
55 | pl_tsystemref | Time System Reference | |
56 | pl_imppar | Impact Parameter | The sky-projected distance between the center of the stellar disc and the center of the planet disc at conjunction, normalized by the stellar radius. |
57 | pl_occdep | Occultation Depth | Depth of occultation of secondary eclipse |
58 | pl_ratdor | Planet-Star Distance over Star Radius | The distance between the planet and the star at mid-transit divided by the stellar radius. For the case of zero orbital eccentricity, the distance at mid-transit is the semi-major axis of the planetary orbit. |
59 | pl_ratror | Planet-Star Radius Ratio | The planet radius divided by the stellar radius |
60 | pl_def_refname | Default Reference | Reference for publication used for default parameter |
61 | pl_disc | Year of Discovery | Year the planet was discovered |
62 | pl_disc_refname | Discovery Reference | Reference name for discovery publication |
63 | pl_locale | Discovery Locale | Location of observation of planet discovery (Ground or Space) |
64 | pl_telescope | Discovery Telescope | Name of telescope of planet discovery observations |
65 | pl_instrument | Discovery Instrument | Name of instrument of planet discovery observations |
66 | pl_status | Status | Status of the planet (1 = announced, 2 = submitted, 3 = accepted, 0 = retracted). |
67 | pl_mnum | Number of Moons in System | Number of moons detected in the planetary system. |
68 | pl_st_npar | Number of Stellar and Planet Parameters | Number of Stellar and Planet Parameters |
69 | pl_st_nref | Number of Stellar and Planet References | Number of Stellar and Planet References |
70 | pl_pelink | Link to Exoplanet Encyclopaedia | It links to the planet page in the Exoplanet Encyclopaedia. |
71 | pl_edelink | Link to Exoplanet Data Explorer | It links to the planet page in Exoplanet Data Explorer. |
72 | pl_publ_date | Publication Date | Publication Date of the planet discovery referee publication. |
74 | hd_name | HD Name | Name of the star as given by the Henry Draper Catalog. |
75 | hip_name | HIP Name | Name of the star as given by the Hipparcos Catalog. |
76 | st_rah | RA (hours) | Right Ascension of the planetary system in decimal hours. |
77 | st_glon | Galactic Longitude (deg) | Galactic longitude of the planetary system in units of decimal degrees. |
78 | st_glat | Galactic Latitude (deg) | Galactic latitude of the planetary system in units of decimal degrees. |
79 | st_elon | Ecliptic Longitude (deg) | Ecliptic longitude of the planetary system in units of decimal degrees. |
80 | st_elat | Ecliptic Latitude (deg) | Ecliptic latitude of the planetary system in units of decimal degrees. |
81 | st_plx | Parallax (mas) | Difference in the angular position of a star as measured at two opposite positions within the Earth's orbit. |
82 | gaia_plx | GaiaParallax [mas] | GaiaDR2 difference in the angular position of a star as measured at two opposite positions within the Earth's orbit. |
83 | st_pmra | RA Proper Motion (mas/yr) | Angular change in right ascension over time as seen from the center of mass of the Solar System. |
84 | st_pmdec | Dec Proper Motion (mas/yr) | Angular change in declination over time as seen from the center of mass of the Solar System. |
85 | st_pm | Total Proper Motion (mas/yr) | Angular change in position over time as seen from the center of mass of the Solar System. |
86 | gaia_pmra | GaiaProper Motion (RA) [mas/yr] | GaiaDR2 angular change in right ascension over time as seen from the center of mass of the Solar System. |
87 | gaia_pmdec | GaiaProper Motion (Dec) [mas/yr] | GaiaDR2 angular change in declination over time as seen from the center of mass of the Solar System. |
88 | gaia_pm | GaiaTotal Proper Motion [mas/yr] | GaiaDR2 total proper motion computed from the RA and Dec. |
89 | st_radv | Radial Velocity (km/sec) | Velocity of the star in the direction of the line of sight. |
90 | st_spstr | Spectral Type | Classification of the star based on their spectral characteristics following the Morgan-Keenan system. |
91 | st_logg | Stellar Surface Gravity | Gravitational acceleration experienced at the stellar surface. |
92 | st_lum | Stellar Luminosity [log(solar)] | Amount of energy emitted by a star per unit time, measured in units of solar luminosities. |
93 | st_dens | Stellar Density [g/cm**3] | Amount of mass per unit of volume of the star. |
94 | st_metfe | Stellar Metallicity (dex) | Measurement of the metal content of the photosphere of the star as compared to the hydrogen content. |
95 | st_metratio | Metallicity Ratio | Ratio for the Metallicity Value ([Fe/H] denotes iron abundance, [M/H] refers to a general metal content) |
96 | st_age | Stellar Age [Gyr] | The age of the host star. |
97 | st_vsini | Rotational Velocity v*sin(i) [km/s] | Rotational velocity at the equator of the star multiplied by the sine of the inclination. |
98 | st_acts | Stellar Activity Index (S-Index) | Chromospheric activity as measured by the S-index (ratio of the emission of the H and K Ca lines to that in nearby continuum). |
99 | st_actr | Stellar Activity Log (R'HK) | Chromospheric activity as measured by the log(R' HK) index, with is based on the S-index, but excludes the photospheric component in the Ca lines. |
100 | st_actlx | x-ray Activity (Lx)" | Stellar activity as measured by the total luminosity in X-rays. |
101 | swasp_id | SWASP Identifier | Name of the star as given by the SuperWASP (Wide Angle Search for Planets) project. |
102 | st_nts | Number of Time Series | Number of literature time series available for this star in the NASA Exoplanet Archive |
103 | st_nplc | Number of Planet Transit Light Curves | Number of literature transit light curves available for this star in the NASA Exoplanet Archive. |
104 | st_nglc | Number of General Light Curves | Number of Hipparcos light curves available for this star in the NASA Exoplanet Archive. |
105 | st_nrvc | Number of Radial Velocity Time Series | Number of literature radial velocity curves available for this star in the NASA Exoplanet Archive. |
106 | st_naxa | Number of Amateur Light Curves | Number of literature amateur light curves available for this star in the NASA Exoplanet Archive. |
107 | st_nimg | Number of Images | Number of literature images available for this star in the NASA Exoplanet Archive. |
108 | st_nspec | Number of Spectra | Number of literature of spectra available for this star in the NASA Exoplanet Archive. |
110 | st_uj | U-band (Johnson) [mag] | Brightness of the host star as measured using the U (Johnson) band in units of magnitudes. |
111 | st_vj | V-band (Johnson) [mag] | Brightness of the host star as measured using the V (Johnson) band in units of magnitudes. |
112 | st_bj | B-band (Johnson) [mag] | Brightness of the host star as measured using the B (Johnson) band in units of magnitudes. |
113 | st_rc | R-band (Cousins) [mag] | Brightness of the host star as measured using the R (Cousins) band in units of magnitudes. |
114 | st_ic | I-band (Cousins) [mag] | Brightness of the host star as measured using the I (Cousins) band in units of magnitudes. |
115 | st_j | J-band (2MASS) [mag] | Brightness of the host star as measured using the J (2MASS) band in units of magnitudes. |
116 | st_h | H-band (2MASS) [mag] | Brightness of the host star as measured using the H (2MASS) band in units of magnitudes. |
117 | st_k | Ks-band (2MASS) [mag] | Brightness of the host star as measured using the K (2MASS) band in units of magnitudes. |
118 | st_wise1 | WISE 3.4um [mag] | Brightness of the host star as measured using the 3.4um (WISE) band in units of magnitudes. |
119 | st_wise2 | WISE 4.6um [mag] | Brightness of the host star as measured using the 4.6um (WISE) band in units of magnitudes. |
120 | st_wise3 | WISE 12.um [mag] | Brightness of the host star as measured using the 12.um (WISE) band in units of magnitudes. |
121 | st_wise4 | WISE 22.um [mag] | Brightness of the host star as measured using the 22.um (WISE) band in units of magnitudes. |
122 | st_irac1 | IRAC 3.6um [mag] | Brightness of the host star as measured using the 3.6um (IRAC) band in units of magnitudes. |
123 | st_irac2 | IRAC 4.5um [mag] | Brightness of the host star as measured using the 4.5um (IRAC) band in units of magnitudes. |
124 | st_irac3 | IRAC 5.8um [mag] | Brightness of the host star as measured using the 5.8um (IRAC) band in units of magnitudes. |
125 | st_irac4 | IRAC 8.0um [mag] | Brightness of the host star as measured using the 8.0um (IRAC) band in units of magnitudes. |
126 | st_mips1 | MIPS 24um [mag] | Brightness of the host star as measured using the 24um (MIPS) band in units of magnitudes. |
127 | st_mips2 | MIPS 70um [mag] | Brightness of the host star as measured using the 70um (MIPS) band in units of magnitudes. |
128 | st_mips3 | MIPS 160um [mag] | Brightness of the host star as measured using the 160um (MIPS) band in units of magnitudes. |
129 | st_iras1 | IRAS 12um [Jy] | Brightness of the host star as measured using the 12um (IRAS) band in units of Jy. |
130 | st_iras2 | IRAS 25um [Jy] | Brightness of the host star as measured using the 25um (IRAS) band in units of Jy. |
131 | st_iras3 | IRAS 60um [Jy] | Brightness of the host star as measured using the 60um (IRAS) band in units of Jy. |
132 | st_iras4 | IRAS 100um [Jy] | Brightness of the host star as measured using the 100um (IRAS) band in units of Jy. |
133 | st_photn | Number of Photometry Measurements | Number of photometry measurements available for this star in the NASA Exoplanet Archive. |
135 | st_umbj | U-B (Johnson) [mag] | Color of the star as measured by the difference between U and B (Johnson) bands. |
136 | st_bmvj | B-V (Johnson) [mag] | Color of the star as measured by the difference between B and V (Johnson) bands. |
137 | st_vjmic | V-I (Johnson-Cousins) [mag] | Color of the star as measured by the difference between V (Johnson) and I (Cousins) bands. |
138 | st_vjmrc | V-R (Johnson-Cousins) [mag] | Color of the star as measured by the difference between V (Johnson) and R (Cousins) bands. |
139 | st_jmh2 | J-H (2MASS) [mag] | Color of the star as measured by the difference between J and H (2MASS) bands. |
140 | st_hmk2 | H-Ks (2MASS) [mag] | Color of the star as measured by the difference between H and K (2MASS) bands. |
141 | st_jmk2 | J-Ks (2MASS) [mag] | Color of the star as measured by the difference between K and K (2MASS) bands. |
142 | st_bmy | b-y (Stromgren) [mag] | Color of the star as measured by the difference between b and y (Stromgren) bands. |
143 | st_m1 | m1 (Stromgren) [mag] | Color of the star as measured by the m1 (Stromgren) system. |
144 | st_c1 | c1 (Stromgren) [mag] | Color of the star as measured by the c1 (Stromgren) system. |
145 | st_colorn | Number of Color Measurements | Number of color measurements available for this star in the NASA Exoplanet Archive. |
147 |
Monday, 1 July 2019
Visualizing confirmed exoplanet detection with Tableau.
Every human child has at one point in their lives dreamed of venturing to the stars and exploring other worlds. For some of those children those dreams manifest themselves in the form trance music played in cave on the southern hemisphere during the longest night of the year, the winter solstice.
For others it manifests as a desire to pursue a career in radio astronomy. I'm on the path of the latter.
Here is proof:
![]() |
Can't stop this Sagan in the makin. Radio astronomy is awesome. |
The search for Exoplanets.
In the last 20 years humanity has begun an incredible preliminary exploration of nearby solar systems that may contain worlds in orbit around other stars.
Using data visualization software Tableau Public as part of my semester break project I have compiled some interesting perspectives on the variety of confirmed exoplanets that exist outside our solar systems, thus producing a series of info-graphics I call the "Infographica Galactica" which is a play on words on the "Encyclopedia Galactica"
This blog post details the development of this project.
Now, radio astronomy and planetary science are two different disciplines. One deals with light at low frequency and the other deals with wandering bodies in orbit around stars but both are connected by the practice of data mining and the art of knowledge discovery and that is exactly the type of approach that will be taken in this project.
*literature review on the planets.
The search for planets beyond our solar system goes hand in hand with the search for extraterrestrial life. So far we only have one model to go on to study the emergence of life in the entire Universe and that is planet Earth in our own solar system. (The verdicts on Mars and Europa have yet to be decided). Therefore, the search for exoplanets boils down to the quest of looking for planets that are "Earth Size" and "Earth like"
We must then establish the distinction between planets that are "Earth Size" and "Earth Like"
NASA Exoplanet Database. the 4,000 worlds
NASA keeps an database archive of confirmed exoplanets. So far there have been 4,000 worlds known without a doubt to exist beyond our solar system. A full list of the planets can be downloaded which makes for great exercise in data mining depending on what you are looking for.
There is separate table available that details the definition of each parameter in the confirmed planets table:
https://exoplanetarchive.ipac.caltech.edu/cgi-bin/TblView/nph-tblView?app=ExoTbls&config=planets
There is separate table available that details the definition of each attribute in the confirmed planets table:
As part of literature review I need to understand what each term does and whether it has any significance for visualization. There are 5 tables detailing terms on the confirmed exoplanet table. Each table corresponds to a different characteristic.
- Default Column
- Planet Column
- Stellar Column
- Photometry Column
- Colour Column
I could do it manually by copying each term from the website into the excel table that I have on my laptop. But as any good programmer knows:
"Anything you have to do repetitively more than 3 times can be automated."
So I scraped it using Beautiful Soup. A cursory glance at the terms shows the following
The table can be found here: http://scienceepicyt.blogspot.com/2019/07/database-column-name-table-label.html
Code is available on github: https://github.com/coderXmachina2
The Draft of my Infographica
Wednesday, 15 May 2019
Tuesday, 7 May 2019
Hippo Poo Vital for Health of East Africa's Rivers
Hippo poo vital for ecosystem balance
There was a piece of News recently about how hippo feces acted as a vital carrier of Nutrients for rivers in East Africa. The large (and adorable) land fauna would eat copious amount of grass and just chill out with the herd in communal hippo pools where they would excrete the grass they had eaten.
The hippo feces contains a large amount of Silicon extracted from the grass that is vital to the health of tiny bacteria called diatoms that live further downstream all the way to Lake Victoria, the largest lake in Africa. This tiny bacteria forms the foundational basis of the diet and food chain of the Mara River in Africa and other rivers that flow into Lake Victoria.
The diatom bacteria are also responsible for carbon sequestration in the environment, that is the act of removing CO2 from the environment. CO2 is a greenhouse gas. Something that if we have too much of in the atmosphere... we die.
I shit you not
Without a thriving bacterial community the environmental balance in the area would be disastrously upset. Fish all along the food chain can't get their nutrition and a dangerous type of life-suffocating bacteria called cyanobacteria will grow to excess causing the emergence of environmental dead zones. That sounds scary.
In the light of recent catastrophes in nearby Mozambique it paints a bleak picture of the future.
The hippo population has suffered a decrease in the last decade and the numbers are projected to further drop but the actions we carry out on now will decide the fate of the hippos and the Mara River. There is still time to stop the key from turning. Once it turns there is no turning back.
It makes me think about this little image here. Credits to Annis Pratt who led me to it.
And the line from the Fourth Hindu Veda:
Whatever I dig from you, O Earth
May your mantle grow back again quickly
O Earth, Purifier, may we never injure you
Sincerely,
SonOfTerra92
![]() |
I have good shit |
There was a piece of News recently about how hippo feces acted as a vital carrier of Nutrients for rivers in East Africa. The large (and adorable) land fauna would eat copious amount of grass and just chill out with the herd in communal hippo pools where they would excrete the grass they had eaten.
The hippo feces contains a large amount of Silicon extracted from the grass that is vital to the health of tiny bacteria called diatoms that live further downstream all the way to Lake Victoria, the largest lake in Africa. This tiny bacteria forms the foundational basis of the diet and food chain of the Mara River in Africa and other rivers that flow into Lake Victoria.
The diatom bacteria are also responsible for carbon sequestration in the environment, that is the act of removing CO2 from the environment. CO2 is a greenhouse gas. Something that if we have too much of in the atmosphere... we die.
I shit you not
![]() |
Hippo Poo makes the Mara River go round |
Without a thriving bacterial community the environmental balance in the area would be disastrously upset. Fish all along the food chain can't get their nutrition and a dangerous type of life-suffocating bacteria called cyanobacteria will grow to excess causing the emergence of environmental dead zones. That sounds scary.
In the light of recent catastrophes in nearby Mozambique it paints a bleak picture of the future.
The hippo population has suffered a decrease in the last decade and the numbers are projected to further drop but the actions we carry out on now will decide the fate of the hippos and the Mara River. There is still time to stop the key from turning. Once it turns there is no turning back.
![]() |
DIatoms in a Psychedelic Universe. We are all connected |
And the line from the Fourth Hindu Veda:
Whatever I dig from you, O Earth
May your mantle grow back again quickly
O Earth, Purifier, may we never injure you
Sincerely,
SonOfTerra92
Saturday, 27 April 2019
Why should we bother looking for Gravitational Waves?
26/4/2019 mid sem Holiday is almost up. I Better get started on that ASTR 800 (Advanced Topics in Astrophysics) paper.
So I want to talk about Gravitational Waves, more so the importance of finding gravitational waves.
Why do we need to find these elusive ripples of propagating space time called Gravitational Waves?
For a long time Gravitational Waves seemed to be secluded within the realm of theoretical physics. The idea was born out of the mind of Einstein while the experimentalists in the room which included Richard Feynman and Joe Weber could only dream of detecting them. Some of those early experimentalists although daring in the quest for gravitational waves are no longer with us, were not able to make it to witness the progress we have made today.
But the first Ligo Gravitational Wave observation happened in 2016 and we can now pinpoint their origins to Supermassive Black Hole Binaries (SMBHB) and Neutron Star Binaries (NSB). Wherever there are really dense astronomical objects orbiting each other in an inspriling cosmic death dance. That is where the origin of gravitational waves can be pinpointed to.
But why do Astronomers have a vested interest in detecting these gravitational waves?
Well that is an interesting question actually. I mean are not observations in the EM domain enough to learn us all we can about the Universe that we live in and the Cosmos from which we spring?
Well, I can tell you that the answer to that is No. Because of something called Multi Messenger Astronomy.
We live in the age of Mutli-Messenger Astronomy (MMA) which is this idea that we can acquire much more information about the universe by looking at signals that reach us in different ways. Here are the 4 ways that information about the Universe can reach us.
Some of things out there may be detectable by the many different media, others in singularly different ones. but there is a wealth of information that can be conveyed by analyzing the same cosmological phenomena in the different messenger media.
![]() |
Break is over, better get started on that term paper.
|
Why do we need to find these elusive ripples of propagating space time called Gravitational Waves?
For a long time Gravitational Waves seemed to be secluded within the realm of theoretical physics. The idea was born out of the mind of Einstein while the experimentalists in the room which included Richard Feynman and Joe Weber could only dream of detecting them. Some of those early experimentalists although daring in the quest for gravitational waves are no longer with us, were not able to make it to witness the progress we have made today.
But the first Ligo Gravitational Wave observation happened in 2016 and we can now pinpoint their origins to Supermassive Black Hole Binaries (SMBHB) and Neutron Star Binaries (NSB). Wherever there are really dense astronomical objects orbiting each other in an inspriling cosmic death dance. That is where the origin of gravitational waves can be pinpointed to.
But why do Astronomers have a vested interest in detecting these gravitational waves?
Well that is an interesting question actually. I mean are not observations in the EM domain enough to learn us all we can about the Universe that we live in and the Cosmos from which we spring?
Well, I can tell you that the answer to that is No. Because of something called Multi Messenger Astronomy.
We live in the age of Mutli-Messenger Astronomy (MMA) which is this idea that we can acquire much more information about the universe by looking at signals that reach us in different ways. Here are the 4 ways that information about the Universe can reach us.
- EM - Light. The classic Medium of Discovery. From Planets to Pulsars this is how its been done since back in the day.
- Gravitational Waves - Ripples of Propagating space time. These can tell us about the merging of really dense objects in the Universe. Black Holes and Netutron Stars
- Neutrinos - Elusive Tiny Particles travelling at the speed of light.
- Cosmic Rays - High Energy Particles. Can tell us about Gamma Ray bursts
Some of things out there may be detectable by the many different media, others in singularly different ones. but there is a wealth of information that can be conveyed by analyzing the same cosmological phenomena in the different messenger media.
One example is how the Gravitational Waves detected by LIGO are quickly followed up by observations by Radio Telescopes. etc.
But what really convinced me about MMA surrounds some discoveries that happened around the time of my birth (1992). That is the discovery of PSR B 1257 + 12 and the planets that surround it. Draugr, Poltergeist and Phobetor.
![]() |
I wonder if it will have an atmosphere. Not that we really need one if we ever get to explore it. I figure we'll carry our own atmosphere with us. |
These 3 planets orbit the husk of a star that went supernova a long time ago. That star is survived today as a pulsar that periodically emits beams of electromagnetic radiation The planets were discovered by measuring the variation in Doppler shift of pulsation Period.
This planet marks the discovery of an extrasolar planet via means of Pulsar Timing. One of the worlds was discovered to be twice the size of the moon at 0.02 Earth Mass, the smallest planet ever to be discovered. In all the planets discovered by the Kepler mission in the modern age. Pulsar Timing in 1992 (the year that I was born) revealed to us a world in a category of its own. A tiny little speck orbiting a Pulsar.
That remarkable discovery was made via an indirect observation by studying subtle changes in the propagation of light. we didn't see the planet optically but implied its existence by looking at the effect it had on the pulsar signal, thus confirming its existence.
Now extend that to Gravitational Waves and the information they carry, and the things we might come to learn of when we detect Grav Waves. What new wonders undreamt of in our own time would will we discover by studying gravitational waves?
Lets find out...
Tuesday, 16 April 2019
AGNs and Cross Matching Algorithms
17/4/2019 Cross Matching in a nutshell.
The solution to this problem is: Cross Matching Algorithms
Here is a diagram of what cross matching implies:
This is what a naive cross matcher (pseudocode) would look like:
for l in range (0, len(cat A)):
for m in range (0, len(cat B)):
calculate offset = angulardistance (A, B)
if offset < radius
if offset = smallest value so far
return best_match = (A, B, offset)
Where A, B are RA, Dec values for a catalog.
These have a connection to big data, and to the whole Billions and Billions thing in the Universe. I will talk about in following entries.
We begin our story with Active Galactic Nuclei colloquially known as AGN. In a nutshell they are Supermassive Black Holes surrounded by accretion disks of matter that shoot out jets of radiation in the form of radio lobes that are pretty exotic things to see in the radio spectrum.
Here is an artists rendition:
They are primordial objects found in the early universe. To borrow a quote from Armand Delsemme "When the Quazars shone their dazzling brilliance"... that "dazzling brilliance" refers to the fact that AGNs can shine at much higher than normal luminosity in all over the EM spectrum.
And in fact they do.
The radiation from the accretion disk is brightest in Optical and Ultraviolet
The Gas and Dust that surrounds the Black Hole is visible in the Infrared
Hot gas surrounding the black hole gets heated up and emits X Rays
The jets emitted by the black hole are visible for many lightyears in radio frequencies
So we point many different telescopes in the same direction in order to see them. There's a diverse ecosystem of telescopes and eyes on the sky that humanity has at its disposal. All of which can be used to study Active Galactic Nuclei
But there is a problem. y and X-ray telescopes are located in space and orbit the Earth with different periodicity. Optical Telescopes are placed on mountain tops all over the world, and radio telescopes are situated in barren deserts tucked far away from any radio frequency interference.
How do we study the same brilliant dazzling objects with such different equipment?
Coordinating these instruments to look at the same object in the sky require a herculean task of cooperation and cross border collaboration that demonstrate capacity for humans to let go of their differences and unite in the effort of studying the Cosmos, ancient and vast from which we spring.
How do we study the same brilliant dazzling objects with such different equipment?
Coordinating these instruments to look at the same object in the sky require a herculean task of cooperation and cross border collaboration that demonstrate capacity for humans to let go of their differences and unite in the effort of studying the Cosmos, ancient and vast from which we spring.
The solution to this problem is: Cross Matching Algorithms
Here is a diagram of what cross matching implies:
![]() |
Cross Matching Astronomical Objects from different Catalogs. VLA, SDDS, Hubble |
for l in range (0, len(cat A)):
for m in range (0, len(cat B)):
calculate offset = angulardistance (A, B)
if offset < radius
if offset = smallest value so far
return best_match = (A, B, offset)
Where A, B are RA, Dec values for a catalog.
These have a connection to big data, and to the whole Billions and Billions thing in the Universe. I will talk about in following entries.
Thursday, 11 April 2019
Matlab FFT on Sinusoidal Code
amp = 3;
freq = 5;
phi = pi/4;
fs = 50;
Ts = 1/fs;
l = 1; %second samples
sineWaveFunc(amp,freq,phi,Ts,l)
plot(t, s)
xlabel('time')
ylabel('Amplitude')
title('sineWave')
myPow = calcPower(fs, freq, s);
myFFT = findFFT(s);
%calculate power
N = length(s) + 1; % to be even :)
S = fft(s,N);
SdB = 20*log10(S); % or SdB = mag2db(S);
freqs = (0:N/2-1)*fs/N;
figure(2)
plot(freqs, SdB(1:N/2))
xlabel('frequency')
ylabel('amplitude')
title('spectrum')
function sinFFT = findFFT(s)
N = length(s) + 1;
sinFFT = fft(s, N);
%plot(sinFFT, N)
end
function pow = calcPower(fs, freq,s)
Nsamp = round(fs/freq);
pow = (1/Nsamp)*sum(abs(s(1:Nsamp)).^2);
end
function [s , t] = sineWaveFunc(amp,freq,phi,Ts,l)
t = 0:Ts:l;
s = amp*sin(2*pi*freq*t + phi);
end
freq = 5;
phi = pi/4;
fs = 50;
Ts = 1/fs;
l = 1; %second samples
sineWaveFunc(amp,freq,phi,Ts,l)
plot(t, s)
xlabel('time')
ylabel('Amplitude')
title('sineWave')
myPow = calcPower(fs, freq, s);
myFFT = findFFT(s);
%calculate power
N = length(s) + 1; % to be even :)
S = fft(s,N);
SdB = 20*log10(S); % or SdB = mag2db(S);
freqs = (0:N/2-1)*fs/N;
figure(2)
plot(freqs, SdB(1:N/2))
xlabel('frequency')
ylabel('amplitude')
title('spectrum')
function sinFFT = findFFT(s)
N = length(s) + 1;
sinFFT = fft(s, N);
%plot(sinFFT, N)
end
function pow = calcPower(fs, freq,s)
Nsamp = round(fs/freq);
pow = (1/Nsamp)*sum(abs(s(1:Nsamp)).^2);
end
function [s , t] = sineWaveFunc(amp,freq,phi,Ts,l)
t = 0:Ts:l;
s = amp*sin(2*pi*freq*t + phi);
end
Tuesday, 9 April 2019
DSP and Astronomy
10/3/2019 Astronomy = (DSP + Statistics + Data Science)
Look at this slide about windowing from DSP Guru Mitra
So that is what this massive phallic construct does:
Which can be broken down into geek speak like this:
So if you have the infinities of a pulsar signal (That's artist embellishment right there) and apply all those DeDispersions, FFTs, phase binning. Then we can get a limited sequence called a power spectrum in terms of frequency and time.
Or we can use it to blow each other up and all that other planetary chauvinism stuff.
Look at this slide about windowing from DSP Guru Mitra
Sequence/Pulse Windowing is the act of turning a infinite length sequence into a finite length sequence by applying a window sequence
So that is what this massive phallic construct does:
Which can be broken down into geek speak like this:
Radio Telescopes are windows on the Universe
So if you have the infinities of a pulsar signal (That's artist embellishment right there) and apply all those DeDispersions, FFTs, phase binning. Then we can get a limited sequence called a power spectrum in terms of frequency and time.
Its just a windowed sequence of values |
Or we can use it to blow each other up and all that other planetary chauvinism stuff.
Monday, 1 April 2019
Matlab Discrete Sinusoid
2/4/2019 Creating Discrete Sinusoidal
I'm going to go learn Cyclic Spectroscopy for Radio Astronomy Applications. Got distracted, plotted Sinusoids in Matlab instead.
a = -80;
b = 80;
n = a:b; %sample length
fs = 0.0125;
phi = 0;
Amp = 6;
x = Amp*sin(2*pi*fs*n - phi);
x2 = (-Amp)*x
clf
stem(n, x, '-o')
axis([0 b -2 2]);
grid;
title('sinusoid')
xlabel('Time index n')
ylabel('Amplitude')
axis
%plotting an evil twin
hold on
stem(n, x2, '-*')
I'm going to go learn Cyclic Spectroscopy for Radio Astronomy Applications. Got distracted, plotted Sinusoids in Matlab instead.
![]() |
I think it looks pretty |
a = -80;
b = 80;
n = a:b; %sample length
fs = 0.0125;
phi = 0;
Amp = 6;
x = Amp*sin(2*pi*fs*n - phi);
x2 = (-Amp)*x
clf
stem(n, x, '-o')
axis([0 b -2 2]);
grid;
title('sinusoid')
xlabel('Time index n')
ylabel('Amplitude')
axis
%plotting an evil twin
hold on
stem(n, x2, '-*')
Saturday, 30 March 2019
Knowledge Discovery with WEKA
30/3/2019 Introduction to WEKA. The Waikato Environment for Knowledge Analysis
The hype for all this AI stuff is getting severely overblown. But that being said predictive algorithms will be an important tool for scientists in any field in the years to come.
Astronomy is no different.
Neural Networks have been used to classify pulsar candidates in this paper:
- V. Morello, E. D. Barr, M. Bailes, C. M. Flynn, E. F. Keane: “SPINN: a straightforward machine learning solution to the pulsar candidate selection problem”, 2014; arXiv:1406.3627. DOI: 10.1093/mnras/stu1188.
Astronomy datasets are notorious for putting the BIG in BIG Data. Not only are the objects being studied are separated from Earth by large distances but also, the true nature of the signals that they produce are magnificently intense.
The paper states that it as the data mounts up in the future, it will no longer be feasible to process it using a typical 'human cogitator' i.e. Grad Students approach to vet the data. That's where the Data Mining and Machine Learning will comes in.
![]() |
Twinkle Twinkle little Pulsar, But is that what you really are? |
So i guess it is important for yours truly to get to grips with it. Thats where WEKA comes in.
WEKA is an an environment that can be used to analyze datasets using prebuilt data mining algorithms.
WEKA is an an environment that can be used to analyze datasets using prebuilt data mining algorithms.
We can test the same data set with different data mining algorithms to take a measure of their performance. This is done by finding their percentage of correctly classified and their time taken to build model.
The following is a description of the Classifier/ Data Mining Algorithm/ Learner that we use to analyze the attributes/feature and put the data into class.
Data mining’s eclectic nature fostered this inconsistency in naming—the field encompasses contributions from statistics, artificial intelligence (Machine Learning),and database management;each field has chosen different names for the same concept.
Data mining’s eclectic nature fostered this inconsistency in naming—the field encompasses contributions from statistics, artificial intelligence (Machine Learning),and database management;each field has chosen different names for the same concept.
One-R (One Rule
Naive Bayes (probabilistic Classifier with strong independence assumption)
IBK (K Nearest Neighbour)
J48 (unpruned C4.5 decision tree)
Random Forest (Random Decision Forests)
MLP (Multi Layer Perceptron)
SMO (Support Vector Machines)
Data-set
|
Classifier
|
Percent
correct (%)
|
Time
to Construct (s)
|
Weather-nominal
|
One-R
|
42.8571
|
0.00
|
Naïve
Bayes
|
57.1429
|
0.00
|
|
IBK
|
57.1429
|
0.00
|
|
J48
| 50.000 |
0.00
|
|
Random
Forest
|
71.4286
|
0.02
|
|
MLP
|
71.4386
|
0.03
|
|
SMO
|
64.2857
|
0.01
|
|
Iris
|
One-R
|
92.0000
|
0.01
|
Naïve
Bayes
|
96.0000
|
0.01
|
|
IBK
|
95.3333
|
0.00
|
|
J48
|
96.0000
|
00.0
|
|
Random
Forest
|
95.3333
|
0.06
|
|
MLP
|
97.3333
|
0.14
|
|
SMO
|
96.0000
|
0.04
|
|
Pima Diabetes
|
One-R
|
65.1042
|
0.00
|
Naïve
Bayes
|
76.3021
|
0.005
|
|
IBK
|
70.1823
|
0.00
|
|
J48
(with missing values)
|
73.8281
|
0.02
|
|
J48 (Remove Corrupt Instances) J48 (Padded Corrupt Instances) | 74.6228 74.0885 |
0.01
0.01 |
|
Random
Forest
|
75.7813
|
0.18
|
|
MLP
|
75.3906
|
0.40
|
|
SMO
|
77.3438
|
0.03
|
|
Soybean
|
One-R
|
39.9707
|
0.01
|
Naïve
Bayes
|
92.9722
|
0.01
|
|
IBK
|
91.215
|
0.00
|
|
J48
|
91.5081
|
0.04
|
|
Random
Forest
|
92.9722
|
0.19
|
|
MLP
|
93.4114
|
20.00
|
|
SMO
|
93.8507
|
0.49
|
Repeating the model building resulted in faster build time but no changes in accuracy.
Support Vector Machine can be not bad
Subscribe to:
Posts (Atom)
Diaries of an Aspiring Astrophysicist (DAS Astro) Podcast
Diaries of an Aspiring Astrophysicist Episode 1: The last year has been weird Episode 2: Cosmic Collisions and Gravitational Wa...
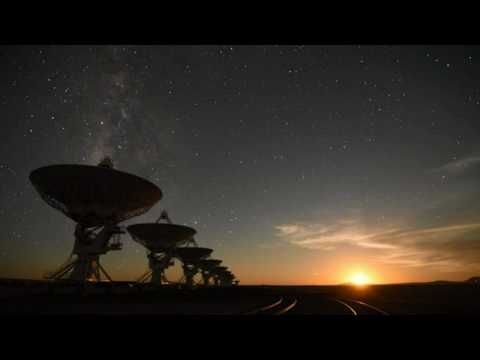
-
How Pulsar Timing Arrays Work Best Explanation so far for how a Pulsar Timing Array Works, and it makes it look more analogous to an Inter...
-
O Brave New World I would like to take some time to talk about what many would consider to be a fringe science science. If you...
-
Lets get started with a really interesting picture ... Now do I have your attention ... Today is yesterdays Tomorrow We live in...